Research
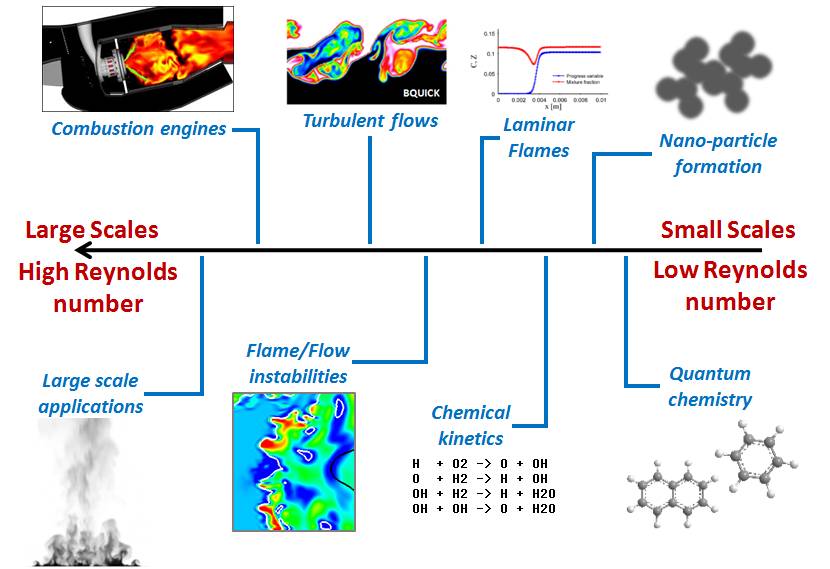
Thermal ignition and flame propagation
Accidental ignition of flammable gases is a critical safety concern in many industrial applications. Particularly in the aviation industry, the main areas of concern on an aircraft are the fuel tank and adjoining regions, where spilled fuel has a high likelihood of creating a flammable mixture. To this end, a fundamental understanding of the ignition phenomenon is necessary in order to develop more accurate test methods and standards as a means of designing safer air vehicles.
The focus of this work is thermal auto-ignition, hot surface ignition, and flame propagation. In this project, we improve continuously the state-of-the-art in hydrocarbon chemistry as it pertains to fuel tank safety; and we develop various tabulation approaches to implement efficiently and yet accurately this chemistry in CFD codes. We consider three different applications: ignition by a hot surface, flame propagation in a temperature-stratified mixture, and ignition by a flying metal spark. In the course of the project, we discovered an unexpected phenomenon: puffing premixed flames. This last phenomenon is a perfect example of the strong coupling between hydrodynamics (buoyancy) and combustion.
Sponsored by the Boeing Company.
Understanding and modeling soot formation
The aim of this project is to fill the gap in the present understanding and modeling of soot formation both in laminar and turbulent flames. The project focuses on the combustion of surrogate fuels comprised of several chemical species in contrast to current single-species models. Our current state-of-the-art chemical model is extended with additional components often found in surrogates. The inner structure of soot particles is investigated as a mean to gain insight into soot inception, growth, and oxidation via the development of a nested hierarchy approach combining Quantum Chemistry, Molecular Dynamics, and Monte Carlo simulations.
The newly improved chemical and soot models is incorporated in Direct Numerical Simulations of turbulent sooting flames and validated in turbulent jet diffusion flames. The outcome is expected to be a major leap in the development of predictive models for the combustion of transportation fuels, the formation/oxidation of soot, and Large Eddy Simulations. The impact of the proposed research goes far beyond soot formation. It is relevant to the formation of all other nanoparticles (SiO2, TiO2, and AlO3) as well as other slowly evolving processes in turbulent flows (CO, NOx).
Sponsored by the US Department of the Energy (Early Career Award).
Turbulent premixed combustion
The objective of the proposed work is to analyze the tight two-way coupling between turbulence and combustion in premixed flames under conditions of relevance to the Air Force, namely at high Reynolds numbers and high Karlovitz numbers. The focus is placed on re-evaluating commonly-made model assumptions that are currently the limiting aspect towards the development of truly predictive simulation tools for Large Eddy Simulations (LES).
The goal of the present work is to perform true Direct Numerical Simulations of both chemistry and turbulence to avoid any model assumptions. The combustion of a kerosene surrogate is represented with a semi-detailed chemical model. The analysis of the chemistry tabulation and the study of flame-turbulence interactions provides the necessary knowledge to assess the validity of current state-of-the-art models for LES and ultimately to improve them.
The outcome is expected to be a more profound understanding of the complex two-way coupling between turbulence and combustion under conditions of relevance to the Air Force and a major leap in the development of predictive models for LES of realistic engine/burner configurations.
Sponsored by the US Air Force Office of Scientific Research.
Turbulent buoyant flows
Rayleigh-Taylor instabilities appear when a heavy fluid is placed on top of a light fluid. The light fluid rises as a result of buoyancy forces, and in many cases, the flow transitions rapidly to turbulence. Due to their intrinsic nature, Rayleigh-Taylor instabilities are found in a large diversity of engineering applications and natural phenomena (inertial confinement fusion, furnaces, accidental fires, heat transfer within stars, supernova formation, underwater hot vents, oil spill...). A lot of work has been done on the initial and long term growth of Rayleigh-Taylor instabilities. However, little is known about the structure of the turbulence inside buoyant mixing layers. A perfect example is the observed anisotropy of scales in direct contradiction with the well established Kolmogorov theory and which remains currently unexplained by any theoretical models.
In order to gain access to the fine details of turbulence, Direct Numerical Simulations of various "controlled experiments" are performed. These configurations span all regimes of turbulent buoyant flows, from weak buoyancy to buoyancy-dominated turbulence. The data are used to analyze the growth of Rayleigh-Taylor instabilities, the emergence of scale anisotropy in buoyant flows, and the effects of Richardson and Atwood numbers. The outcome is multiple and includes the formulation of a novel theoretical framework unifying turbulent non-buoyant and buoyant flows. The project will result also in the development of new subgrid scale models specifically designed for Large Eddy Simulations of turbulent buoyant flows.
Sponsored by the National Science Foundation (NSF Career Award).
High Schmidt number turbulent mixing
Passive scalars are used to represent the concentration of chemicals, different fluids, and particulates. They are characterized by their Schmidt number (the ratio of gas viscosity to scalar diffusivity). The interaction between convection, diffusion, and turbulent transport leads to the formation of small scale structures, which become increasingly small as the Schmidt number increases. Turbulent mixing is important in a wide variety of scientific and engineering applications, such as the environmental dispersion of a saline solution or dye, sediment transport, ocean temperature distribution, flow visualization...
High quality experiments and numerical simulations have been done already. Unfortunately, disparities exist between experimental observations and numerical results. The generally-accepted, theoretical analysis from Batchelor has not yet been fully validated. This is due to the lack of overlap between experiments (performed at high Reynolds and Schmidt numbers) and simulations (limited to one or the other).
The overall research plan relies on two simple observations: 1) scalar fluctuations are much smaller than velocity fluctuations, and 2) scalar fluctuations are controlled by the turbulent velocity field. The current project takes advantage of this scale separation (inherent to high Schmidt turbulent flows) to develop new simulation tools to investigate both high Reynolds and high Schmidt number flows.
Hypersonic gas-surface interactions
The study of gas-surface interactions and material ablation encountered during hypersonic flight is at the crossroads of several scientific disciplines, and thus requires a wide range of expertise including aerodynamics, kinetics of non-equilibrium flows, surface chemistry, and material sciences. Moreover, it necessitates the combination of experiments and numerical simulations.
In collaboration with the T5 shock tunnel facility at Caltech, we propose to perform fully integrated numerical simulations of the flow/chemistry encountered during hypersonic flight. The objective is to incorporate the various developments in chemical models into a unique CFD framework for hypersonic flows.Beyond simple validation and verification, these numerical simulations will guide the experimental work by suggesting new materials, shapes, and operating conditions to investigate. Furthermore, this framework will be used to analyze potentially new concepts for smart ablators.
 |